Focus On 'Gravitational Waves and Massive Black Holes'
Image 1:
Ground-based telescope view (left) of the collision between the galaxies NGC4038 and NGC4039, which reveals long arcing insect-like ‘antennae’ of luminous matter flung from the scene of the accident. Investigations using the Hubble Space Telescope have revealed over a thousand bright young clusters of stars – the result of a burst of star formation triggerd by the collision. The green outline shows the area covered by the higher resolution Hubble image (right). Dust clouds around the two galactic nuclei give them a dimmed and reddened appearance, while the massive, hot young stars of the new formed clusters are blue.
Though they have not been directly detected so far, gravitational waves are a necessary consequence of Einstein’s theory of General Relativity. They distort space and time, changing the perceived distances between free macroscopic bodies. A gravitational wave passing through the Solar System creates a time-varying strain in space that periodically changes the distances between all bodies in the Solar System in a direction that is perpendicular to that of wave propagation. These could be the distances between spacecraft and the Earth, as in the case of Ulysses or Cassini (attempts were and will be made to measure these distance fluctuations), or the distances between shielded ‘proof masses’ inside spacecraft that are separated by a large distance, as in the case of LISA.
The collaborative NASA/ESA Laser Interferometer Space Antenna (LISA) mission, planned for launch in 2012, will be the first space mission to search for these elusive gravitational waves. As the technology needed for the project is highly demanding, a precursor technology-demonstration mission is considered to be a necessary pre-requisite. Called LISA Pathfinder (formerly SMART-2) and planned for launch in 2008, it was given the go-ahead on 7 June by ESA’s Science Programme Committee (SPC).
What Are Gravitational Waves ?

Image 2:
Stellar motions in the vicinity of Sgr A. The orbital accelerations of stars close to the Galactic Centre have been studied with near-infrared high-resolution observations. The resulting data allow contraints to be placed on the position and mass of the central supermassive black hole.
In Newton’s gravitational theory, the gravitational interaction between two bodies is instantaneous, but according to Special Relativity this should be impossible because the speed of light represents the limiting speed for all interactions. So, if a body changes its shape the resulting change in the force field should make its way outwards no faster than the speed of light.
Einstein's paper on gravitational waves was published in 1916, and that was about all that was heard on the subject for over forty years. It was not until the late 1950s that some relativity theorists, and in particular Herman Bondi (before he became the first Director General of ESRO, ESA’s forerunner), proved rigorously that gravitational radiation was in fact a physically observable phenomenon, that gravitational waves carry energy, and that, as a result, a system that emits gravitational waves should lose energy.
Einstein’s Theory of General Relativity replaces the Newtonian picture of gravitation by a geometric one that is very intuitive, if we are willing to accept the fact that space and time do not have an independent existence, but rather are in intense interaction with the physical world. Massive bodies then produce ‘indentations’ in the fabric of ‘spacetime’, and other bodies move in this curved spacetime taking the shortest path, much like a system of billiard balls crossing a spongy surface. In fact, Einstein’s equations formally relate mass (energy) and spacetime curvature in just the same way that Hooke's law relates force and spring deformation. Put more poignantly, spacetime is an elastic medium and if a mass distribution moves in an asymmetric way, then the spacetime indentations travel outwards as ripples in spacetime called ‘gravitational waves’.
Gravitational waves are fundamentally different from the familiar electromagnetic waves: the latter are created by the acceleration of electric charges and propagate in the framework of space and time, whereas gravitational waves are created by the acceleration of masses and are waves of the spacetime fabric itself. As early as 1805, Laplace, in his famous Traité de Mécanique Céleste stated that, if gravitation propagates with a finite speed, the force in a binary star system should not point along the line connecting the stars, and the angular momentum of the system must slowly decrease with time. Today we would say that this happens because the binary star is losing energy and angular momentum by emitting gravitational waves. 188 years later, in 1993, Hulse and Taylor were awarded the Nobel Prize for Physics for their indirect proof of the existence of gravitational waves using exactly the kind of observation that Laplace had suggested, on the binary pulsar PSR 1913+16. However, the direct detection of gravitational waves has still not been achieved.
Gravitational waves are only very weakly coupled to matter and, therefore, suffer negligible scattering or absorption. This makes them an ideal cosmological and astrophysical ‘information carrier’, because all of the gravitational waves that have ever been emitted in the Universe are still traveling untouched through space. The main problem is that the ‘straining’ of spacetime, i.e. the fractional change in the distance between masses, due to the passage of a gravitational wave, is exceedingly small. For example, the periodic change in distance between two proof masses, separated by millions of kilometres, due to a typical white dwarf binary at a distance of 50 parsec* is only 10[-10] m. This is not to say that gravitational waves are weak in the sense that they carry little energy. On the contrary, a supernova in a not too distant galaxy will drench every square metre here on Earth with kilowatts of gravitational radiation intensity. The resulting length changes, though, are very small because spacetime is an extremely stiff elastic medium and so it takes extremely large energies to produce even minute distortions.
Where Do Gravitational Waves Come From?
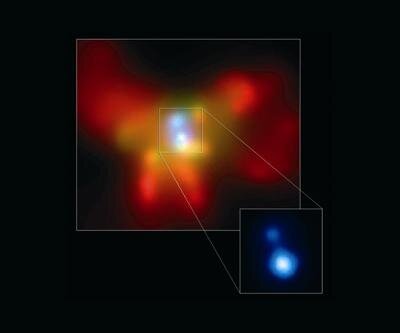
Image 3:
This image, taken by the Chandra spacecraft, show the butterfly-shaped galaxy NGC6240, which is the product of the collision of two smaller galaxies. The central region of the galaxy has two active giant black holes (inset).
The two main categories of sources for the gravitational waves for which LISA will be searching are galactic binary star systems and massive black holes expected to exist in the centres of most galaxies. Because the masses involved in typical binary star systems are small (a few times the mass of the Sun), the observation of binaries is limited to our own Galaxy. Galactic sources that can be detected by LISA include a wide variety of binaries, such as pairs of close white dwarfs, pairs of neutron stars, neutron-star and black-hole (5 to 20 solar masses) binaries, pairs of contacting normal stars, normal-star and white-dwarf (cataclysmic) binaries, and possibly also pairs of black holes. Some galactic binaries are already so well studied that they are considered to be ‘calibration sources’. One such example is the X-ray binary 4U1820-30, located in the globular cluster NGC 6624. If LISA would not detect the gravitational waves from such known binaries with the intensity and polarisation predicted by the Theory of General Relativity, it would shake the very foundations of gravitational physics!
The main objective of the LISA mission, however, is to learn about the formation, growth, space density and surroundings of massive black holes. There is now compelling indirect evidence for the existence of massive black holes with masses 106 to 108 times that of the Sun in the centres of most galaxies, including our own. The most powerful sources are the mergers of massive black holes in distant galaxies. Observations of signals from these sources would test General Relativity and particularly black-hole theory to unprecedented accuracy. Little is currently known about black holes with masses ranging from about 100 to 106 times that of the Sun and LISA can provide unique new information throughout this mass range.
During the gravitational capture of a star by a black hole, gravitational waves will be continuously emitted, allowing an accurate map to be made of the spacetime surrounding the black hole. It will therefore finally be possible to verify whether the ‘black holes have no hair’ theorem* is true.
Read full article (pdf)
Authors:
Alberto Gianolio, Guiseppe Racca
Scientific Projects Department, ESA Directorate of Scientific Programmes
ESTEC, Noordwijk, The Netherlands
Oliver Jennrich, Ruedeger Reinhard
Research and Scientific Support Department, ESA Directorate of Scientific Programmes
ESTEC, Noordwijk, The Netherlands
Karsten Danzmann
Institut für Atom- und Molekülphysik und Albert-Einstein Institut
University of Hannover, Germany
Stefano Vitale
Department of Physics, University of Trento, Italy