Microbial consortia for space biomining
Background
In order to enable durable human presence in space, solutions need to be developed to overcome the problem of limited resources that can be taken from Earth. For the inhabited missions to the Moon and Mars planned in the not-so-distant future [1], the use of in situ resources (ISRU) may be a solution as it will allow sustainable exploration and settlement of space by using resources that are already there, instead of importing them. Among the different approaches such as physical, mechanical, chemical, there is a growing interest in the use of living organisms, such as microorganisms, for ISRU. As they are capable of self-replication and can be versatile and resilient, an approach using them can be self-sustaining with little to no human assistance, as well as being energetically economic compared to other classical approaches [2].
An application of this approach is regolith conversion using the concept of primary succession. A previous ACT study focused on converting the lunar regolith into arable soil using living organisms instead of fertilizers in order to limit resources transported from the Earth. The idea is to use pioneer species, organisms like plants and microoganisms able to colonize new bare habitats. They are usually very resilient as they can develop in hostile environment, and by doing so they contribute to and accelerate the conversion of the bare rock to a soil, allowing for more complex plants to grow.
Mining is another process for which microorganisms can be used. One critical aspect for an extended stay in space is the maintenance of habitats and machines, which indeed require material resources like metals and minerals. Conventional mining methods can be used in space but need a lot of energy and heavy drilling equipment that have to be transported from Earth. Therefore, there is a need for an efficient, self-sustaining and cost-effective mining process, and biomining, the use of microorganisms for metal extraction, is an interesting alternative method. On Earth, biomining is actively used and is for example responsible of more than 20% of copper yearly production in the world [3]. In comparison with the conventional mining methods, biomining is less energy demanding, sustainable and more respectful of the environment [4], which is why there is a growing interest in space biomining [5, 6]. Taking Mars as a use case, is it possible to use biomining to autonomously extract metals in order to prepare human space exploration?
Project summary
![Metal recovered by the biomining of Lunar regolith simulant EAC-1A with the fungus Penicillium simplicissimum. [9]](/gsp/ACT/images/projects/biomining/EAC_exp.jpg)
Different types of microorganisms can be used and among them are filamentous fungi (also known as moulds), a very diverse group of eukaryotic organisms that can form filamentous structures called hyphae. They are heterotrophic organisms and some of which are known to solubilize metals through a mechanism called bioleaching, as well as able to tolerate high levels of metals [7] and to survive in space conditions (space stations, radiations, ...) [8]. A recent study has shown the ability of a fungus, Penicillium simplicissimum, to extract metals from a lunar regolith simulant, EAC-1A, and has shown interesting results such as tolerance to high concentration of regolith, adaptation to gravity, and the recovery of 10 +/- 3 g/L of metal powder [9]. However, as fungi are aerobic and heterotrophic organisms, they need oxygen and organic carbon source, which cannot be found in space. Therefore this makes them non-autonomous, as they need to be provided with the necessary resources in order to grow.
Instead of relying on physical reactions or transport from Earth to provide substrates for the fungi, using other living organisms to do so will keep the biomining process self-sustainable. For the example of oxygen, cyanobacteria are good candidates of organisms able to provide fungi with oxygen via photosynthesis, but as the radiation levels on Mars are higher and the amount of sunlight received on the surface is limited compared to Earth, the photosynthesis activity will be limited. The subsurface of Mars would be a more suitable environment to host the organisms as they would get protection from radiation, but light would be absent and photosynthesis would be impossible. However, a recent study of the groundwater ecosystems of Alberta, Canada, has detected unprecedented levels of oxygen, showing the presence of aerobic metabolic activity in the subsurface ecology [10]. This is explained by the presence of (per)chlorate dismutating bacteria, organisms that are able to produce oxygen out chlorite by an oxydo-reduction reaction [11]. Perchlorate, that can be reduced to chlorite, is also known to be present in large amounts on Mars. These bacteria could then sustain the growth of the fungi, but how these organisms will behave over time?
This project is focusing on the use of biological consortia to enable a self-sustaining artificial ecology composed of bioleaching fungi and oxygen producing bacteria, in the context of Martian biomining. This system is studied by taking a mathematical modelling approach, as it would allow to explore the space of parameters. The system is modelled using ordinary differential equations (ODEs) representing the growth and interactions of the various components through substrates. Model parameters were obtained using literature and reference experimental data, and the evolution and stability of the system was studied using mathematical methods for dynamical systems.
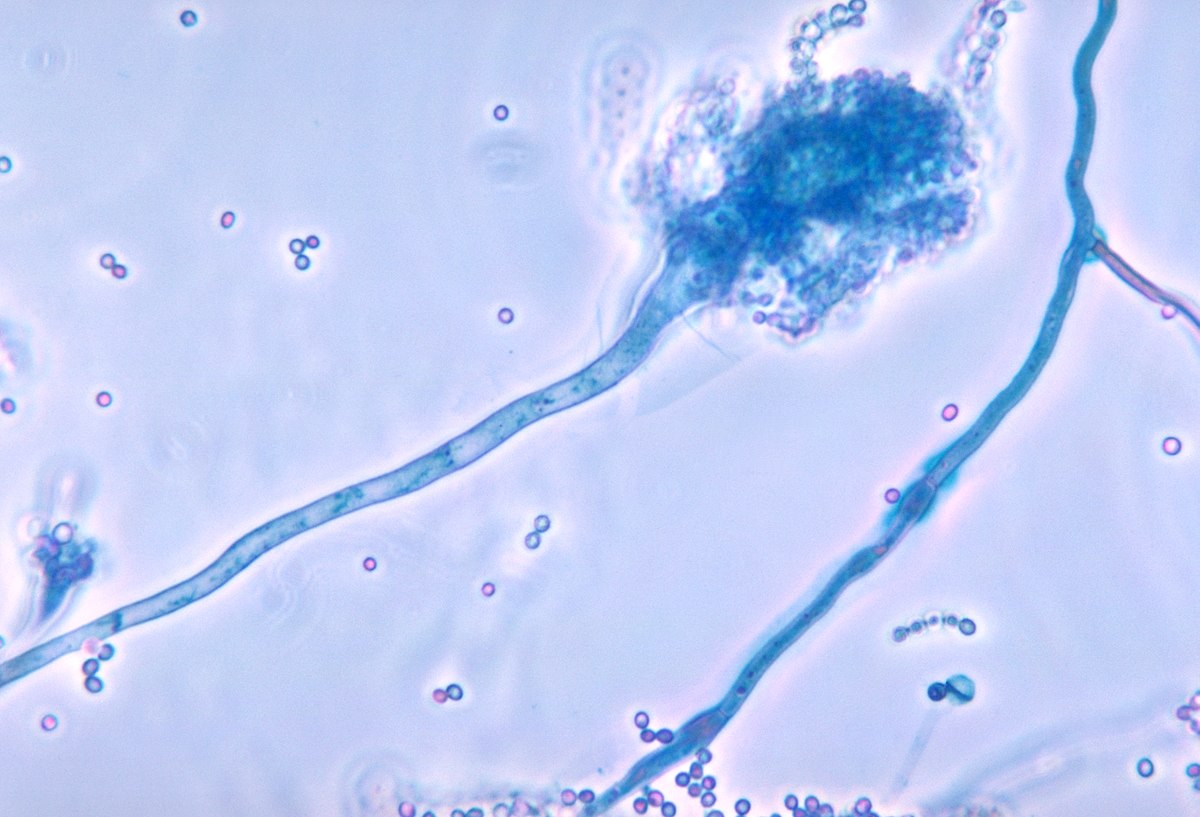
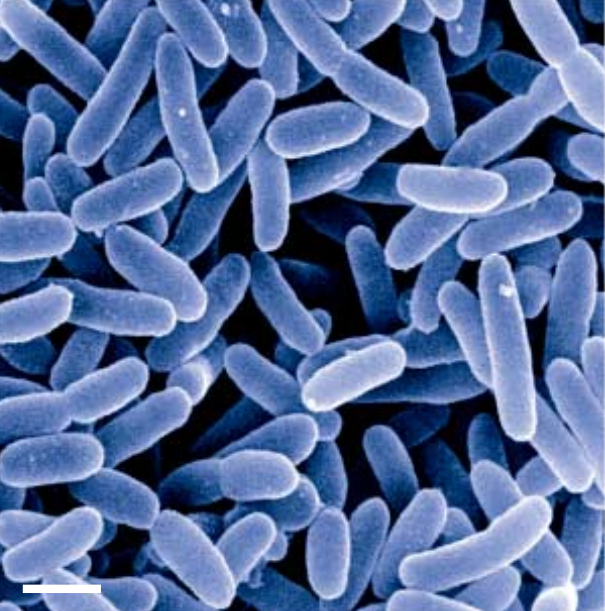
References
[1] Laurini, Kathy, et al. "The global exploration roadmap." IAC-11 B 3 (2018).
[2] Averesch, Nils JH, et al. "Biomanufacturing for Space-Exploration–What to Take and When to Make." (2022).
[3] Johnson, D. Barrie, Barry M. Grail, and Kevin B. Hallberg. "A new direction for biomining: extraction of metals by reductive dissolution of oxidized ores." Minerals 3.1 (2013): 49-58.
[4] Srichandan, Haragobinda, et al. "Bioleaching approach for extraction of metal values from secondary solid wastes: a critical review." Hydrometallurgy 189 (2019): 105122.
[5] Santomartino, Rosa, Luis Zea, and Charles S. Cockell. "The smallest space miners: principles of space biomining." Extremophiles 26.1 (2022): 7.
[6] Gumulya, Yosephine, Luis Zea, and Anna H. Kaksonen. "In situ resource utilisation: the potential for space biomining." Minerals Engineering 176 (2022): 107288.
[7] Anahid, S., S. Yaghmaei, and Z. Ghobadinejad. "Heavy metal tolerance of fungi." Scientia Iranica 18.3 (2011): 502-508.
[8] CortesĂŁo, Marta, et al. "Aspergillus niger spores are highly resistant to space radiation." Frontiers in Microbiology 11 (2020): 528685.
[9] Figueira, Joao, et al. "Biomining of Lunar regolith simulant EAC-1A with the fungus Penicillium simplicissimum." (2023).
[10] Ruff, S. Emil, et al. "Hydrogen and dark oxygen drive microbial productivity in diverse groundwater ecosystems." Nature Communications 14.1 (2023): 3194.
[11] Bardiya, Nirmala, and Jae-Ho Bae. "Dissimilatory perchlorate reduction: a review." Microbiological research 166.4 (2011): 237-254.