Vibrating systems in general relativity
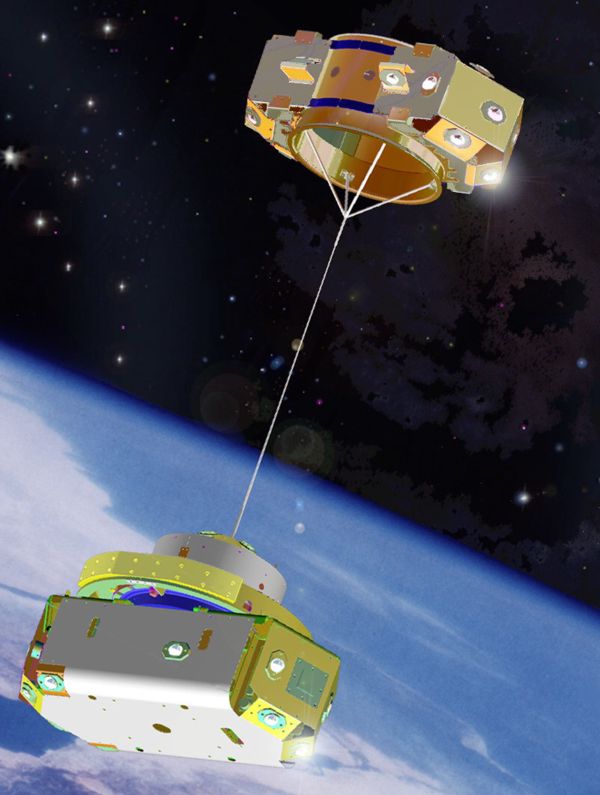
Vibrating systems can have an interesting behavior in general relativity. In this project, simple systems with time dependent constraints are considered. We study the deviation of these vibrating systems from a free fall trajectory.
Propulsion is an important and delicate topic in spacecraft dynamics. Chemical propulsion (ejecting gases) and electrical propulsion (ejecting ions) are widely used, but there exists more advanced concepts such as the use of photons. All these ideas rely on the conservation of momentum of the system, composed by the spacecraft and the propellant. However, the dream of many aerospace engineers is to design a propellantless propulsion. This is possible via an interaction with an external force, which can come from the gravitational field, the magnetic field of a planet, or the solar wind (solar sails). It is also possible to change the angular momentum by internal movements.
In this project, we study a simple system constituted by two masses linked by a massless tether, of which the length changes periodically with time. It has been shown that with a once-per-orbit change of the tether length, it is possible to change the eccentricity and energy of the orbit whilst conserving angular momentum [1,2]. If the frequency of the tether length changes is linked to the spinning frequency of the system, then it is possible to change the angular momentum [3]. However, all these Newtonian effects are limited since they are resonant effects: the change of shape is linked to the motion of the system.
A new way to test general relativity?
What happens now if we consider this problem in the theory of general relativity? Then non-resonant effects appear which can become larger than Newtonian effects [4-6]. The change of the shape of the system is no longer limited, and significant deviations can be obtained from the free fall for high frequency oscillations of the tether. This effect has been studied in [5] and called the relativistic glider [6], as one can use this effect to decelerate the fall of a vibrating system in the gravitational field of a planet.
The first part of this project provided a more detailed analysis of the relativistic glider. We derived an analytical expansion of the dynamical equations, confirming that the effect is proportional to 1/R^2, for radius, R, up to 1000 times the Earth radius; we expect then that it is not possible to see this effect in the gravitational field of the Earth or larger planets. On the other hand, we showed that the deviation from the free fall increases linearly with increasing duration of the fall. It is thus very interesting to increase this duration with, for example, a circular orbit. We also studied the influence of the initial conditions of the system, as well as the influence of different implementations of the constraint [7].
In the second part of this project, we studied the same system in a circular orbit. The equations of motion could be solved analytically with some approximations. We found that the vibrations in the system change the circular orbit to an ellipse (see the video{:target="_blank"}). In general relativity, the dominant contribution to the deviation is a high-velocity effect and the maximal deviation from the circular orbit grows linearly with the radius of the orbit R. This is to be compared with the Newtonian effect which decays like 1/R, therefore, it could be possible to measure this effect in the solar system. We estimated an order of magnitude of the deviation for a semi-classical model of a molecule - in high orbit and for a highly excited molecule, the deviation can attain five centimeters.
References
- M. Martinez-Sanchez and S.A. Gavit, "Orbital modifications using forced tether-length variations"{:target="_blank"}, Journal of Guidance, Control, and Dynamics,Vol. 10, No. 3 (1987), p. 233-241
- G.A. Landis and F.J. Hrach, "Satellite relocation by tether deployment"{:target="_blank"}, Journal of Guidance, Control, and Dynamics, Vol. 14, No. 1 (1991),p. 214-216
- G.A. Landis, "Reactionless orbital propulsion using tether deployment"{:target="_blank"}, Acta Astronautica (1992), Vol. 26, 5, p. 307-312
- J. Wisdom, "Swimming in spacetime: motion by cyclic changes in body shape"{:target="_blank"}, Science (2003), 299, no.5614, p. 1865-1869
- E. Guéron, C.A.S. Maia and G.E.A. Matsas, ""Swimming" versus "swinging" effects in spacetime"{:target="_blank"}, Physial Review D, 73,024020 (2006)
- E. Guéron and R.A. Mosna, "Relativistic glider"{:target="_blank"}, Physical Review D 75, 081501 (2007)
- L. Bergamin, P. Delva and A. Hees, "The relativistic glider revisited"{:target="_blank"}