RObotic GEostationary orbit Restorer (ROGER)
The RObotic Geostationary orbit Restorer (ROGER) studies focussed on the need for and feasibility of a mission to control the threat from faulty satellites and large debris in the geostationary orbit. The geostationary orbit has a high commercial and strategic value and the satellite systems using it for telecommunication, TV broadcasting and weather forecasting represent a significant value in terms of capital investment and revenues. Dependency on the GEO is bound to increase as more basic services (telecommunication, weather forecast, and global navigation) rely heavily on it.
The aim of the work was
- to examine the threat from overcrowding in GEO,
- determine possible mitigation measures (including which assisted re-orbiting),
- subsequently work out a justification for a Robotic Geostationary Orbit Restorer, and
- finally to suggest a plausible technical implementation for such a mission and identify the necessary development and demonstration activities.
The work was carried out under ESA Contracts by two teams in competition:
- ASTRIUM (D) DLR (D), TU Braunschweig (D), MD Robotics (CND) Space Application Services (B), EADS-LV (F) and SES-ASTRA (L)
- QinetiQ (UK), OHB-System (D), ESYS (GB) and Dutch Space (NL)
which provided different solutions to the problem.
The threat
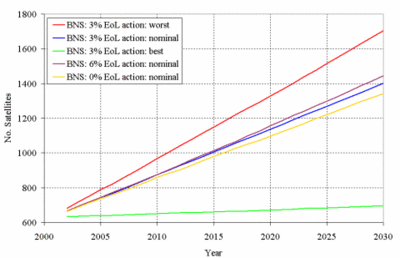
The future growth of space debris has been studied and characterised extensively for low Earth orbit and more recently for the geosynchronous orbital environment. For objects in geostationary or geosynchronous orbits the presence of even a relatively small number of satellites has a special significance given the small range of suitable orbital parameters. The unique operational characteristics of GEO mean that all users are constrained to the same narrow corridor of space. Given that there is no natural sink for objects at GEO altitudes the number of objects in the corridor will continue to increase, enhancing the collision risk, unless some mitigation measures are implemented. As of January 2002 there are 900 reported objects in GEO of which about 28% are controlled, operational satellites Sources of debris in GEO are primarily considered to be uncontrolled satellites that have either exhausted their propellant reserves or have failed, and launch vehicle upper stages. Additionally there is a small contribution from related objects, such as explosive bolts and lanyards, and the two explosive break-ups known to have occurred in GEO. Additional analysis revealed 239 objects in GTO orbit that regularly intersect the operational GEO region, noting that the injection accuracy of launch vehicles could play a significant role in determining the potential hazard such objects pose to the geostationary orbit. Predicting the future to try and understand the full extent of the problem two software tools have been designed to provide an analysis of the effect on the Geostationary ring of current and future satellite operations. The GEO Simulators have been used to investigate the best and worst cases thought to apply in GEO over the period to 2030. For the nominal future satellite population there is about 2.8% chance of a collision occurring between two tracked objects in the GEO region before 2030. However the worst case shows there could be as many as 1700 satellites in the Geostationary orbit by the year 2030. 79% of these could be uncontrollable giving a 3.7% risk of collision, or 1 in 25 chance.
Prevention is better than cure.
Year | Abandoned | Inadequate | Reorbited | Total |
---|---|---|---|---|
1997 | 6 | 5 | 7 | 18 |
1998 | 8 | 6 | 7 | 21 |
1999 | 6 | 2 | 4 | 12 |
2000 | 3 | 3 | 2 | 8 |
2001 | 5 | 7 | 2 | 14 |
Totals | 28 | 23 | 22 | 73 |
In order to preserve this resource for future satellite operations users have been encouraged over the years to re-orbit their satellites at end of life. This involves boosting the satellite to a graveyard region about 300 km above the GEO ring. Today this practice is not fully embraced by all satellite operators, indeed only one third of satellites that reached the end of their missions last year are believed to have successfully re-orbited. At present there are no enforceable regulations on the re-orbiting of satellites. The Inter Agency Space Debris Committee (IADC) has established a guideline for re-orbiting which has been incorporated into the draft European Space Debris Mitigation Standards (EDMS) document. Nearly 40% of all geostationary satellites are not re-orbited at the end of their design lives and only 30% are re-orbited in accordance with the IADC guideline. Approximately 3 – 4% of GEO satellites could not be re-orbited even if the operators were prepared to do so, due to onboard failures.
The case for ROGER
ROGER - the RObotic GEostationary orbit Restorer - is a new concept for an in-orbit roving debris removal system. ROGER can be tasked to approach and capture a redundant or non-operational satellite in the Geostationary (GEO) orbit and tow it into a parking or graveyard orbit.
The ROGER study investigated in detail many different mission scenarios and the mechanics of undertaking the mission. These included:
- Government or commercially funded re-orbiting mission using a minimum development route (e.g. with no reliance on robotics)
- Government or commercially funded re-orbiting using currently mature robotic technology (i.e minimum development still consistent with servicing possibilities)
- Commercially funded servicing mission using currently mature robotic technology
- Commercially funded servicing mission using more advanced technology, expected to be mature in perhaps 10-15 years, aiming to take advantage of a wider range of servicing targets.
The technical challenges were identified as a small number of key design drivers and critical technologies identified for a GEO intervention mission. The most significant issues are the following:
- the ability to safely capture a target satellite is constrained by the target satellite's dynamic behaviour, its shape and structure and the availability of solid capture and docking points,
- the number of target satellites within a multiple target mission is technically limited by the OCS capability in terms of propellant mass and a reasonable spacecraft reliability during mission design lifetime. Consideration should be given to the trade-off of transfer time and propellant mass for high Isp electric propulsion systems,
- the ACS of ROGER has to be capable to control both the ROGER satellite and the tandem "ROGER plus target satellite" within all mission phases,
- the ability to perform the intervention case (iii) and the related event types are constrained by the availability and capability of dextrous robotics,
- the supervision, control and eventually tele-manipulation of ROGER from ground, especially during the close approach, capture and docking, taking into account the data turn-around latency for communication with GEO
The ROGER spacecrafts
Different configurations of the ROGER spacecraft have been identified. These differ not only in the basic satellite bus but mainly in the means used to "capture" a target satellite. The two results are hereafter shown.
ROGER Spacecraft by the ASTRIUM team

The proposed satellite platform is a derivative of a former very detailed designed Astrium platform, with detailed structural and thermal analysis. Most of the foreseen equipment is existing or of-the-shelf equipment, allowing to keep low the platform cost. The communication subsystem equipment is adapted to the special mission requirement, which requires a change of parameters in the link budget and also other components like antennas. Two additional thrusters clusters of 2 x 10 N thrusters each for the special transportation task to transport targets into the graveyard orbit connected by a tether with ROGER. The avionic and propulsion equipment like high pressure tanks, valves and pressure regulators are located on the lower part of ROGER, whereas all equipment of the payload like the capture mechanism and the vision system are located around the upper platform. The solar generator consists of eight body mounted solar array plates between lower and upper platform.
- ROGER launch mass: 3.500 kg
- Propellant mass: 2.700 kg
- Growth potential 600 kg propellant
- Bus power consumption: 300 W
- S-Band TC up-link
- S-Band TM down link
- Video down link rate: 2 Mb/s
- Attitude pointing accuracy <0.25 deg
- Number of 10 N thrusters: 22
- 400 N thruster for Apogee manoeuvre
Roger uses 20 throw-nets to capture its targets. Each Net capture mechanism weighs 9kg. The mechanism has 4 flying weights to pullout the net. Net dimension: 10mx10m/15mx15m. Net storage volume of 5 litres.
- throwing of the net towards the target satellite
- closing of the net around the target satellite
- ROGER pulling the target satellite to the graveyard orbit (GYO)
- the tether cable is severed leaving the satellite stranded in GYO
ROGER Spacecraft by the QinetiQ team
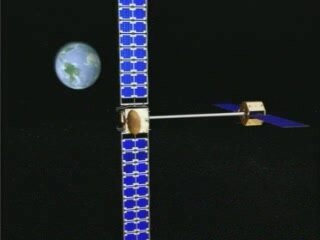
The ROGER spacecraft is based on a new bus design rather than a current commercial GEO spacecraft bus primarily for ease of payload accommodation. It is shaped as an octagonal prism, with the telescopic payload boom mounted on the centre axis of the spacecraft. The solar generators are folded flat onto opposing surfaces of the bus, as are the tentacles of the octopus grappling equipment. The stationary plasma thrusters of the OCS system are mounted on the top surface of the bus.
- Launch Mass:1,450 kg into GTO
- In-Orbit Mass: 1,250 kg wet GEO, 920 kg dry GEO
- Launch Dimensions: 2.7 x 2.7 x 2.9 m3
- Deployed Width: 17 m
- Launcher: Ariane 5 shared launch compatible
- Power: 4,300 W EoL from 32 m2 GaAs Solar Array
- TT&C: S– Band
- Data Downlink: S– Band (2 Mbit/s)
- ACS: 24 mono-propellant hydrazine thrusters
- OCS: 4 SPT electric thrusters for GTO - GEO transfer and re-orbiting
The principal payload element is the grappling equipment. The trade off for grappling equipment has lead to the adoption of a novel bulk capturing device dubbed the "octopus tentacle solution". As a result of further design trades this is now defined by two main structural subsystems:
- Telescopic Boom Subsystem
- Tentacle Subsystem
The Telescopic boom is mounted on the Roger spacecraft and supports the Tentacles which perform the grappling action. At the end of the deployment the tubular segments of the boom are locked to make the joints stiff and to eliminate all undesired play. The end section of the boom is not locked, but spring loaded and allowed to be pushed back into the larger section to guarantee a soft contact with the target-object. The baseline for the Telescopic boom is a telescopic aluminium mast with spring-assisted deployment. There are four tentacles of sufficient reach to encircle any potential target-object and they can be operated simultaneously or independently. The tentacles consist of tapered articulated fingers with a soft surface on the contact side. In the hinges, springs have been added to provide the required local grappling torque, while a number of redundant memory metal wires, which contract when heated electrically, provide the opening torque. In this way loss of contact (grip) during a power drop is prevented and no power is needed during the long towing phase. Once ROGER had reached the target an on-board video system will send images back to the operation centre to help locate the best location for grabbing. During the last phase of approach, the human operator on the ground will not be able to interact with ROGER, because of the time delay in the communication between Ground station and the spacecraft. An automatic short-range distance detection system takes over for the final approach.