High-Speed Experimental Fly Vehicles
HEXAFLY Overview
Motivation
Civil high-speed transportation has always been hampered by the lack of range potential or a too high fuel consumption stemming from a too low cruise efficiency. Indeed, looking into the performance of classically designed high-speed vehicles, their performances drop nearly linearly with flight Mach number. Over the last years, however, radical new vehicle concepts were proposed and conceived having a strong potential to alter this trend. This innovative approach is based upon a well elaborated integration of a highly efficient propulsion unit with a high-lifting vehicle concept. The realization of both a high propulsive and aerodynamic efficiency is based upon the minimization of kinetic jet losses while striving to the best uniformity but minimal induced velocity for lift creation.
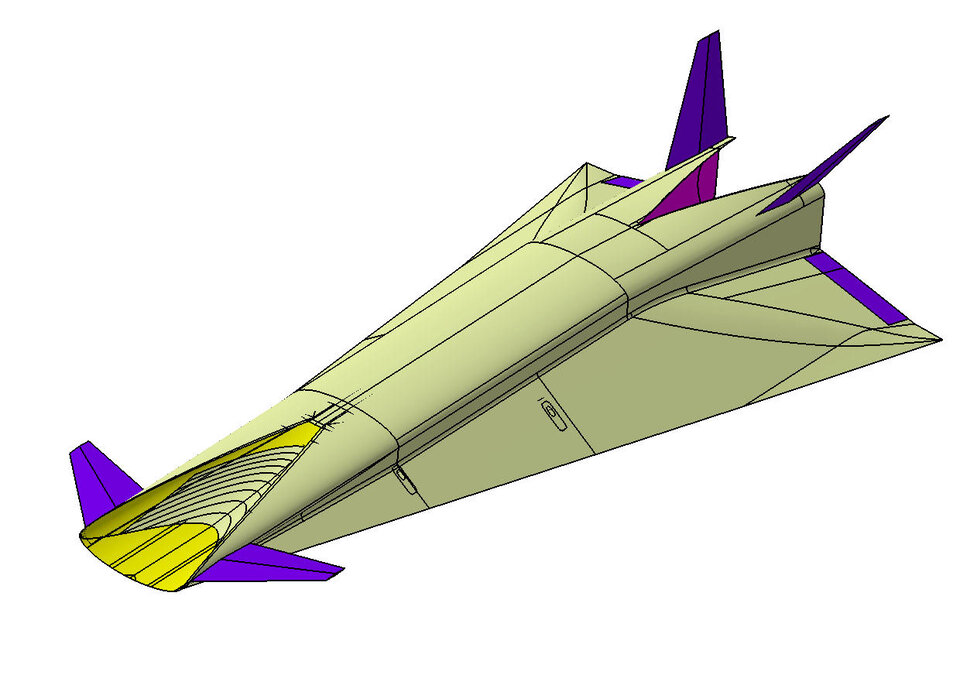
Performing a test flight will be the only and ultimate proof to demonstrate the technical feasibility of these new promising high-speed concepts versus their potential in range and cruise. This would result into a major breakthrough in high-speed flight and create a new era of conceptual vehicle designs.
At present, the promised performances can only be demonstrated by numerical simulations or partly experimentally. As high-speed tunnels are intrinsically limited in size or test duration, it is nearly impossible to fit even modest vehicle planform completely into a tunnel (Fig. 2). Therefore experiments are limited either to the internal propulsive flowpath with combustion but without the presence of high-lifting surfaces, or to complete small-scaled aero-models but without the presence of a combusting propulsion unit. Though numerical simulations are less restrictive in geometrical size, they struggle however with accumulated uncertainties in their modelling of turbulence, chemistry and combustion making complete Nose-to-Tail predictions (Fig. 3) unverified without an in-flight validation. As a consequence, the obtained technology developments are now limited to a technology readiness level of TRL=4 (components validated in laboratory).
Objectives
The HEXAFLY project aims to achieve a first maturation and a proof of concept to experimentally fly-test these radically new conceptual designs accompanied with several breakthrough technologies on board of a high-speed vehicle. This approach would increase drastically the Technology Readiness Level (TRL) up to 6 (System demonstrated in relevant environment).
The emerging technologies and breakthrough methodologies strongly depending on experimental flight testing at high speed can be grouped around the 6 major axes of HEXAFLY:
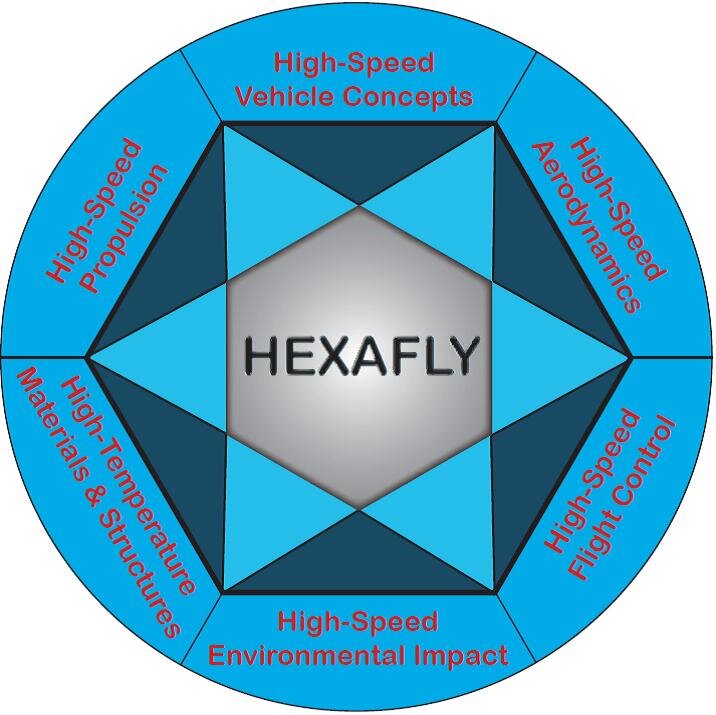
- High-Speed Vehicle Concepts to assess the overall vehicle performance in terms of cruise-efficiency, range potential, aero-propulsive balance, aero-thermal-structural integration, etc...
- High-Speed Aerodynamics to assess e.g. compressibility effects on transition, aerodynamic vehicle shapes with high L/D, stability, etc…
- High-Speed Propulsion to evaluate the performances of high-speed propulsive devices such as intakes, air-breathing engines (ABE), nozzles (SERN) including phenomena such as high-speed combustion, injection-mixing processes, etc…
- High-Temperature Materials and Structures to flight test under realistic conditions high temperature lightweight materials, active/passive cooling concepts, reusability aspects in terms oxidation, fatigue, etc…
- High-Speed Flight Control requiring real-time testing of GNC (Guidance Navigation Control) in combination with HMS/FDI technologies (Health Monitoring Systems/ Fault Detection and Isolation)
- High-Speed Environmental Impact focusing on reduction techniques for sonic boom and sensitivities of high-altitude emissions of H20, CO2, NOx on the stratosphere.
Outline
To mature this experimental flight testing, a scientific mission profile will be defined followed by a proof-of-concept based upon:
- a preliminary design of a high-speed experimental flight vehicle covering the 6 major axes
- selection and integration of the ground-tested technologies developed within LAPCAT I & II, ATLLAS I & II and other national programs
- identification of the most promising flight platform(s)
allowing to address following items:
- identification of potential technological barriers to be covered in a follow-up project
- assessment of the overall ROM-costs to work the project out in a follow-up project
- the progress and potential of technology development at a higher TRL
The vehicle design will be the main driver and challenge in this project. The prime objectives of this experimental high-speed cruise vehicle shall aim for
- an integrated conceptual design demonstrating a combined propulsive and aerodynamic efficiency
- a positive aero-propulsive balance at a cruise Mach number of 7 to 8 in a controlled way
- making optimal use of advanced high-temperature materials and/or structures
- an evaluation of the sonic boom impact by deploying dedicated ground measurement equipment Once conceived, the level of acceleration from Mach 5 up to a cruise speed of Mach 8 can be determined.
The level of complexity and the number of technologies to be potentially integrated largely depends on the affordable size of the flight vehicle. The presently available ground facilities within Europe limit the size to ~1.5m length whereas ~4.5m seems to be an affordable upper limit stemming from presently on-going flight experiments in the US and Russia. Though a larger scale can accommodate a higher density of technology and measurements points, the smaller scale will excel in terms of a lower complexity, weight, size and cost. Therefore, a trade-off is necessary to actually evaluate how the above listed objectives can be realized within an achievable technical and financial scope. Therefore, HEXAFLY is a necessary step prior to embarking onto a larger experimental flight project by identifying, sorting out and alleviating any potential technological risk upfront.
This will require a:
- definition of one preliminary experimental flight vehicle layout but scaled at a small (~1.5m) and a larger scale based upon the available aero- and propulsive databases of the Mach 8 vehicle concept investigated within the LAPCAT II project
- definition of flying test-beds to perform the job: i.e. ground-launched (e.g. sounding rockets) and/or air-launched boosters (e.g. Raduga-D2 booster released from Tu-22 supersonic aircraft):
- sounding rockets for both scales
- air-launched only for the largest scale
- identification of existing flight experimental configurations as a building platform for partly or full integration of a pre-defined vehicle concept
- evaluating how far ground-test results can or need to be extrapolated towards real flight conditions by means of numerical tools and/or ground facilities, e.g. wall temperature effects, scaling…
The databases, simulation tools and methodologies developed within LAPCAT I & II and ATLLAS I & II will be fully exploited to achieve the above listed requirements and to highlight potential technological hurdles. Furthermore, the two proposed scales for the very same vehicle geometry allow assessing the increased density of gauges and inclusion of additional new technologies on the largest vehicle scale versus the technical and financial risks.
Besides the vehicle and platform definition, the suitability of the launch site and test range need to be assessed in terms of potential limitations on the mission and scientific objectives, i.e. flight corridors, TTC (telemetry, tracking and command), safety aspects (termination) and recovery. In a first step, test ranges within Europe and Russia will be favoured. The Andoya test range in Norway, the Esrange and the NEAT range in Sweden are equipped and suitable for ground-launched flight tests in Europe. For air-launch ranges however, no sufficient downrange capability is available within Europe whereas Russia can open some of its test ranges to Europe for experimental flight tests as formerly done for NASA and now for France.
All of the above investigations shall be performed in enough depth to evaluate the ROM-costs for the two flight vehicle scales, their integration and the corresponding launch methodologies. The outcome will provide an overview of the most promising routes towards high-speed flight testing in function of size, complexity and cost. Equipped with these results, the European community on high-speed aircraft will be further aligned and able to profile themselves uniformly towards any international collaboration on flight testing as promoted by the European Commission, in particular Russia, Japan and Australia.