Jupiter’s radiation belts – and how to survive them
ESA’s Jupiter Icy Moons Explorer, Juice, is headed to the largest structure in the Solar System – not the gas giant itself but the mammoth magnetic field that it generates. Its exact size varies with the solar wind, but Jupiter’s magnetosphere is on average 20 million kilometres across, which is about 150 times wider than its parent planet and almost 15 times the diameter of the Sun. But within that field lurks a clear and present danger to space missions – intense belts of radiation much more energetic and intense than Earth’s own Van Allen belts.

Jupiter’s magnetosphere traps charged particles – variously originating from the solar wind or emitted from volcanic Io and other Jovian moons – and then speeds them up, like a terrestrial particle accelerator. The highest fluxes of energetic particles are found in doughnut-shaped belts around Jupiter’s equator, which have been studied by radio astronomers since the 1960s – producing loud bursts of radio noise.
The problem for Juice mission planners is that heading to Jupiter’s icy moons means passing through the radiation belts as well. Europa is the closest orbiting of the three Jupiter’s large ‘Galilean’ moons to be visited by Juice – any human astronaut that lands there would receive a lethal radiation dose on a timescale of hours . And spacecraft electronics are almost as susceptible to radiation as human biology.
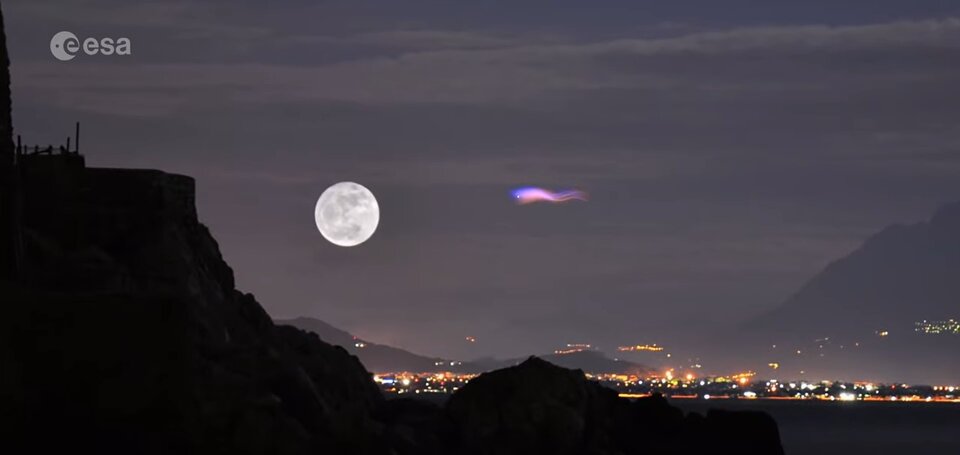
Modelling the danger
“One of the first things we needed was a detailed radiation model for Jupiter space, which could then serve as the basis of mission analysis, and set rad-hardening requirements for Juice’s instruments and components,” explains Christian Erd, Juice spacecraft and system manager.
The mission team turned to ESA’s Space Environment and Effects section, part of the Agency’s Directorate of Technology, Engineering and Quality, who together with other European experts developed the ‘JOvian Specification Environment’, JOSE, calculating fluxes for high-energy electrons and protons around Jupiter.
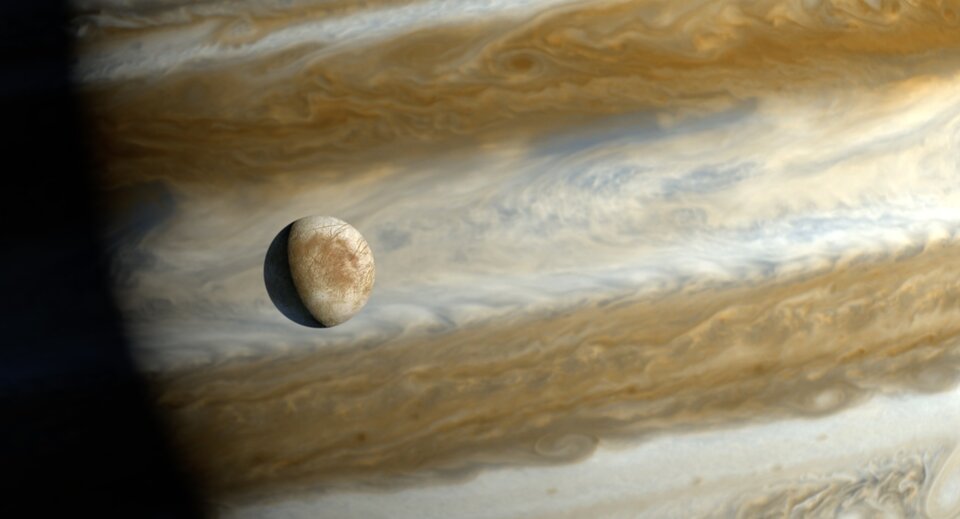
“To build the model we took data from all previous Jupiter missions – Pioneer, Voyager, the Cassini flyby and Galileo in particular, which spent the most time at Jupiter,” notes Petteri Nieminen, overseeing the section. “The Americans had a model, and we put together a European equivalent, largely built on the same data but with some differences in interpretation, ending up with differing values – we revisited instrument modelling analysis for instance.”
The JOSE model is run through ESA’s well-established Space Environment Information System software, SPENVIS, allowing mission planners to generate candidate spacecraft trajectories and assess their resulting radiation exposure. The model includes confidence levels to show how much the figures can be relied on, so appropriate margins can be applied as appropriate to minimise risk.
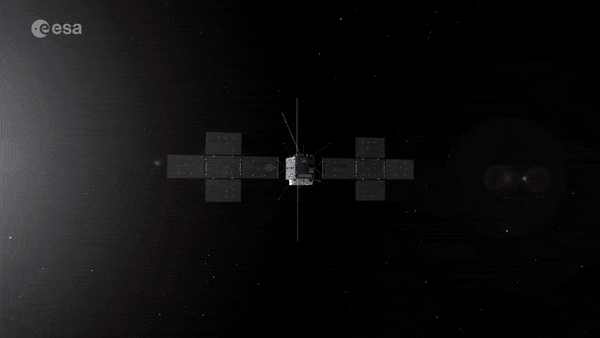
“The model helped shape the detailed mission design, such as the best trajectories to take to minimise radiation damage, including avoiding low latitude orbital paths around Jupiter,” adds modelling specialist Hugh Evans. “The same is true when it came to designing the spacecraft and instruments, including large-scale ‘Monte Carlo’ analysis to study the physics of what would happen when particles interacted with their structure and components.”
Exploration goals in reach
Guided by the JOSE model, Juice will fly past Callisto 21 times, but will only fly past Europa twice – in the process sustaining a third of all its lifetime radiation exposure – before settling into orbit around Ganymede, a moon with its own magnetic field, which works to shield some of Jupiter’s radiation.
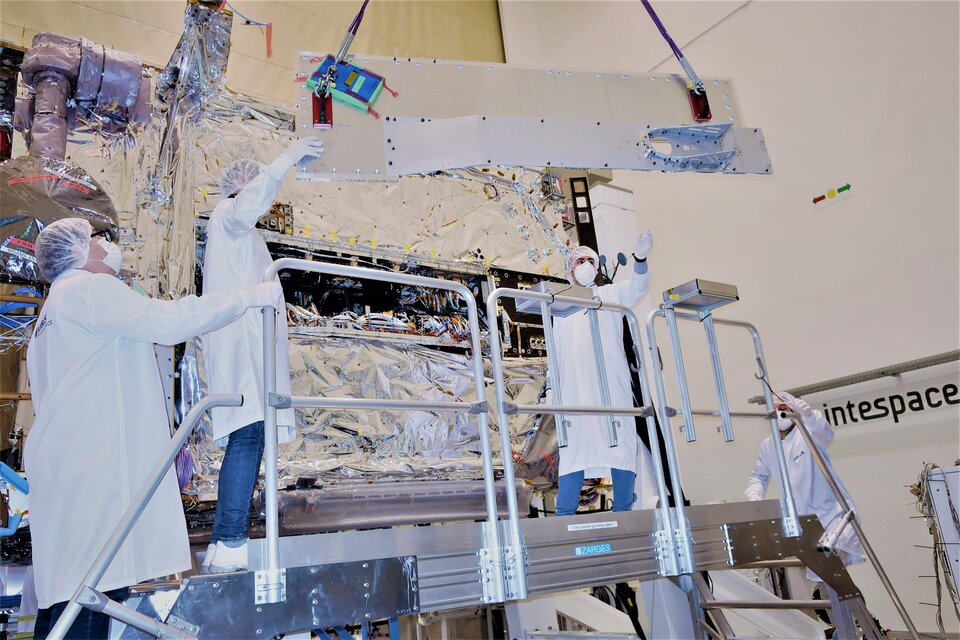
Christian adds: “One of the most important things the model achieved for us was showing that what seemed to be a dangerous place was not completely out of reach. Less than four years at Jupiter will involve the equivalent radiation exposure of a telecommunications satellite in geostationary Earth orbit for 20 years – which we have plenty of experience in managing.”
For added protection, Juice’s most sensitive electronics have been placed inside twin ‘vaults’ within the body of the spacecraft, whose carbon-fibre walls are reinforced with lead. Some components have also been given ‘spot’ shielding made from aluminium or heavier materials such as tantalum.

Time for testing
Even so, all candidate components for the spacecraft platform and its ten instruments had to be carefully selected for their long-term reliability and often laboriously tested for radiation susceptibility, a process overseen by experts from both ESA’s Components section and Radiation Hardness Assurance and Component Analysis section.
“We did the same for Juice that we do for all ESA missions, except that the time was tight and there were a lot of components - because Juice is a huge programme - and the radiation levels were much higher than usual,” comments components engineer Lionel Bonora. “We needed to assess the electronics making up the spacecraft platform, its instruments and the connections in between.”
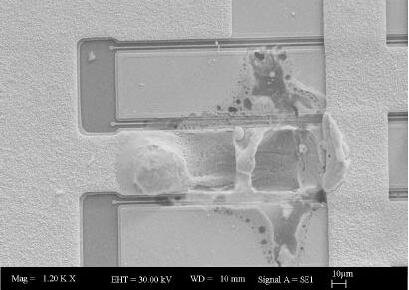
When a charged particle impacts a component, the immediate effect is something called a ‘Single Event Upset’, a brief electrical discharge that might lead to random glitches, memory flips or possibly ‘latch-ups’ – localised short circuits. More significant is the ‘Total Ionising Dose’, which is a gradual degradation of functionality as radiation exposure builds up internal defects within a part.
“Degradation does not always happen slowly, sometimes it can happen quite fast; effects are unpredictable for new technologies,” adds Lionel. “The same phenomenon affects satellites around Earth, but is much less seen on the ground except somewhere like nuclear power stations. The key point is that the components no longer work nominally. There are already components on the market that are made to be radiation tolerant, but that doesn’t include everything we need, so that many units needed testing.”
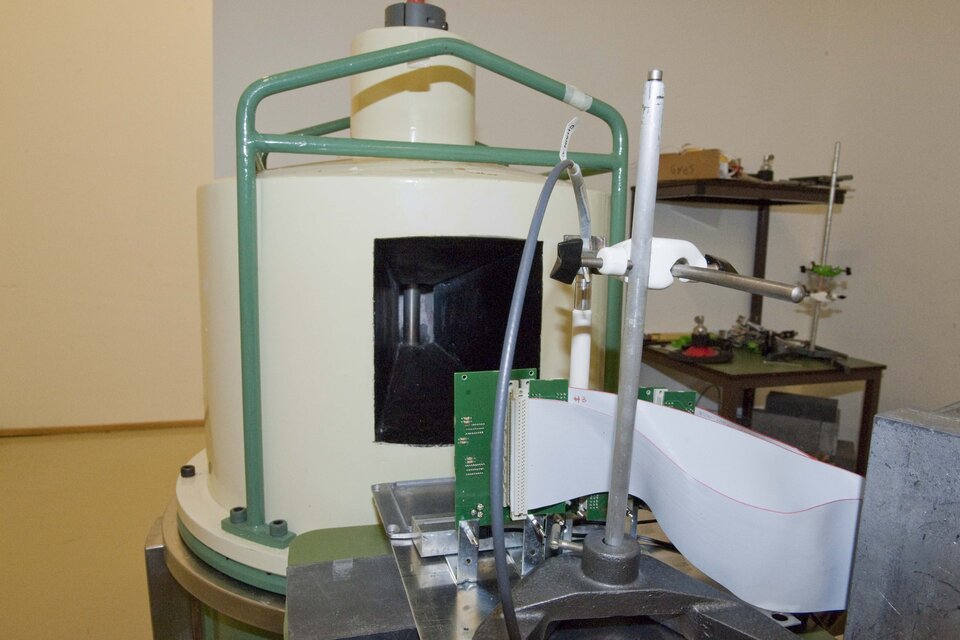
ESA’s ESTEC technical centre in the Netherlands has a gamma ray facility, useful for simulating Total Ionising Dose Effects. Additional radiation testing facilities were employed across Europe for component test campaigns.
From medical support to space testing
Lionel notes: “Most of these 'Single Event Upset' facilities are primarily medical centres, using radiation beams for cancer treatment, and we use their beams when they are free. Depending on the type of effect, we test parts both when they are running and when they are turned off, to take account of redundant parts. We have to do this testing, because space missions are using more and more commercial parts, and there’s no easy way to tell their radiation susceptibility in advance.”
If a part proves to be vulnerable then countermeasures are possible, such as physical shielding or remedies in the software – like error detection, or the so-called ‘triple mode redundancy’ involving one or several chips performing calculations in triplicate then voting for the most likely answer, to reduce radiation-driven disruption. Or in some cases components have to be given up on entirely, and alternatives found.
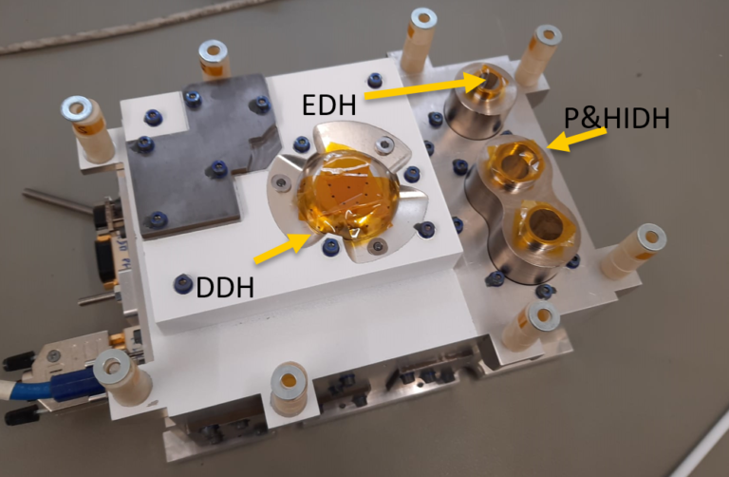
Radiation mapper flying aboard
Once Juice reaches Jupiter, ESA’s Space Radiation and Effects experts are looking forward to finding out how accurate their JOSE model is in practice.
“We have a radiation monitor flying aboard the mission, known as the Radiation-hard Electron Monitor, RADEM, which has been specially designed to observe Jupiter’s high radiation fluxes, equipped with sensors to track how a particle enters and leaves the device,” explains Hugh.
“As soon as the sensor is activated, we plan to cross-check results with other radiation sensors we have placed in orbit, especially during a Solar Particle Event or one of the flybys that Juice makes of Earth en route to Jupiter. Then, when Juice makes it to Jupiter, we’ll get much better data, which will mean in turn that our next radiation model for Jupiter will be even more precise.”